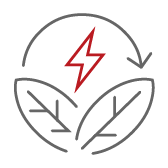
Intelligent, Green Energy for a Better Planet
Predictions
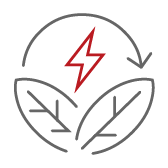
Directions for Exploration
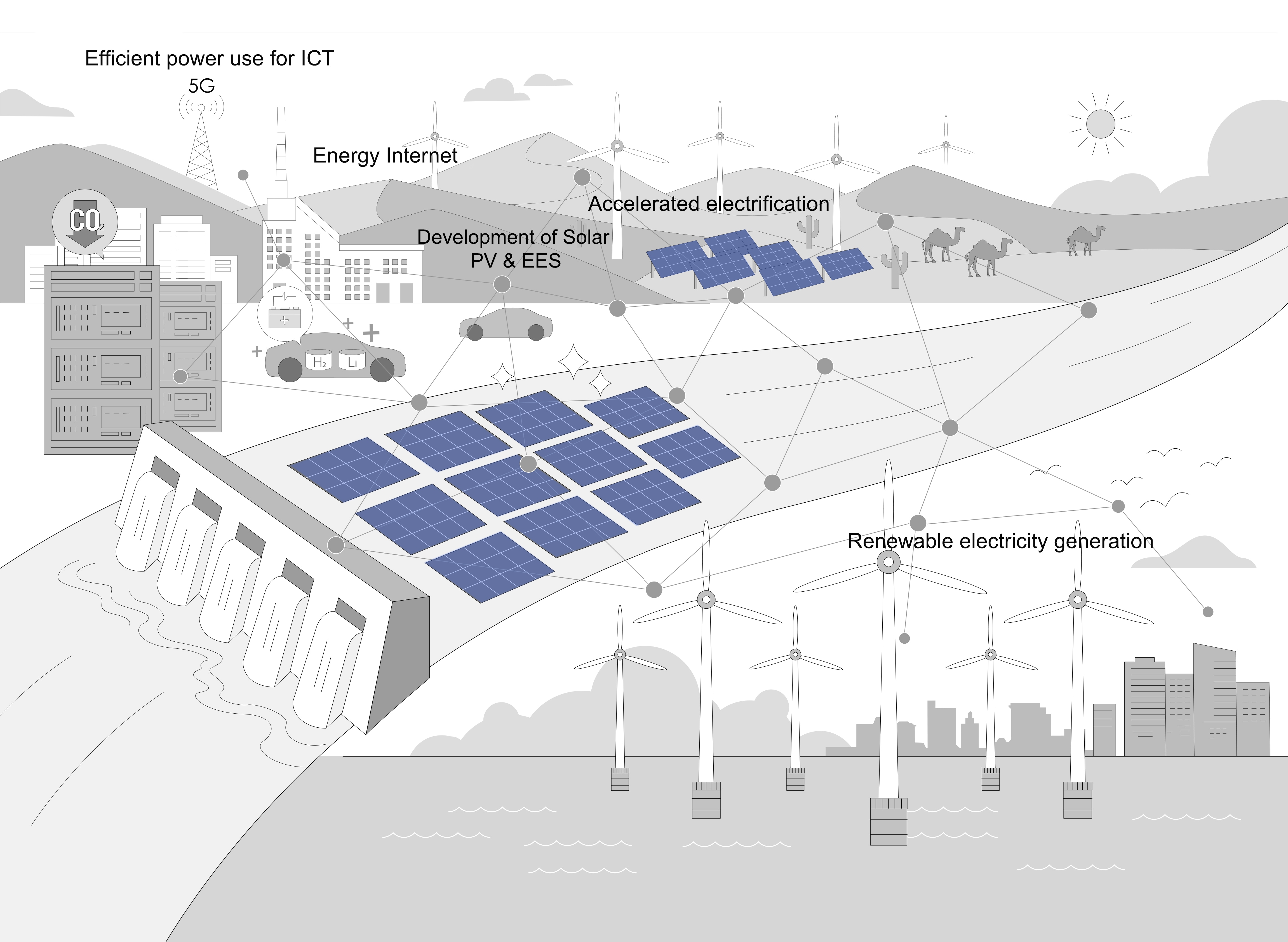
The solar PV and energy storage industries will develop rapidly, expanding from a few countries to the entire world.
Snapshot from the future: Accelerated development in utility-scale, commercial & industrial (C&I), and residential scenarios
Utility-scale power plants achieve economies of scale, reduce unit energy costs, and improve energy utilization through centralized management and optimized energy configuration. Power plants that feature a synergy of wind, solar, hydro, thermal power, storage, and hydrogen are attracting increasing attention.
Technological advances have reduced the levelized cost of electricity (LCOE) for PV power by more than 90%, enabling PV power to achieve grid parity in most regions. The return on investment (ROI) for C&I and residential PV scenarios has been rapidly increasing. Consequently, all-scenario commercialization is becoming the mainstream business model.
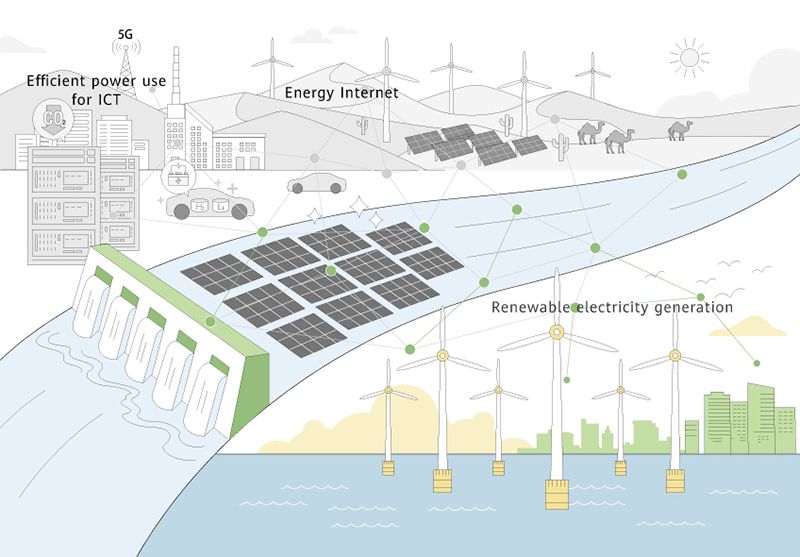
Renewable electricity generation: Floating power plants
Snapshot from the future: Offshore wind and floating PV (FPV) are promising energy sources for the future
Offshore electricity generation can solve challenges that onshore projects confront, such as land shortages, distances from electrical load centers, reduced efficiency of solar PV systems under high temperatures, and biodiversity loss.
On average, wind speeds 10 km offshore are 25% faster than those at the shoreline. Offshore wind turbines can generate power for 3,000 hours annually, compared to 2,000 hours for onshore turbines. By 2030, offshore wind turbines are expected to have rotor diameters of 230–250 meters and capacities of 15–20 MW, which is 3 to 4 times greater than onshore turbines. Floating turbines can be installed in waters up to 60 meters deep. New high-voltage direct current (HVDC) technology offers a cost-effective solution for transmission over distances of 80–150 kilometers from the coast. The Global Wind Energy Council (GWEC) forecasts that global offshore wind capacity will increase from 75 GW today to 275 GW by 2030, with annual installations growing at 25% per year over the next five years.
Compared to land-based PV (LBPV) systems, FPV systems that are installed on water save land. The absence of obstacles on the water surface reduces shading loss and dust buildup. Additionally, the natural cooling effect of the water and higher offshore wind speeds can enhance PV performance. Studies show that FPV systems perform about 12.96% better annually than LBPV systems. The global FPV market capacity is projected to exceed 60 GW by 2030, with an estimated potential capacity of 400 GW worldwide.
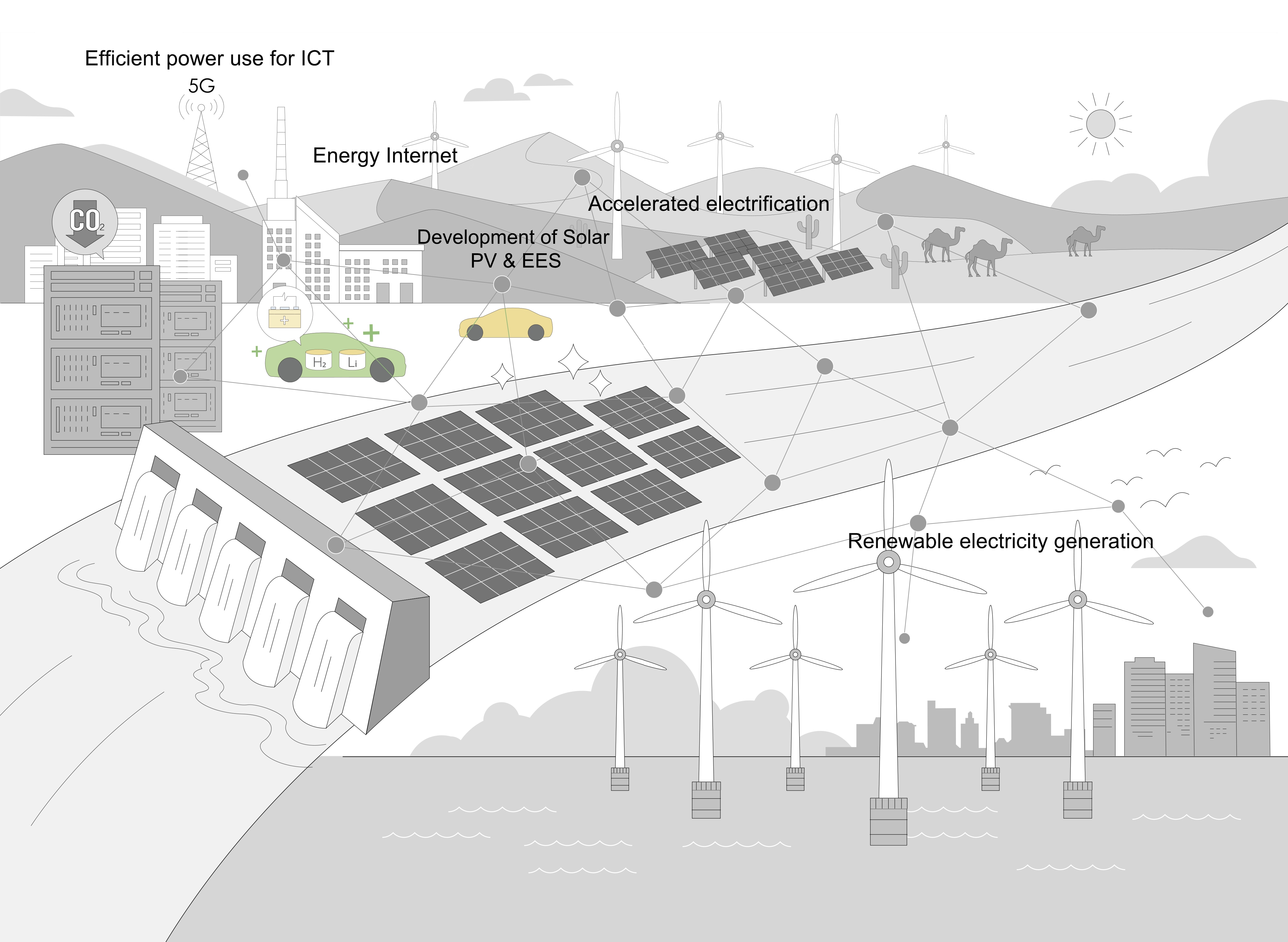
The future energy world will be centered on electricity, and green hydrogen is emerging as a big player.
Snapshot from the future: The adoption of electricity will accelerate across industries, diverse energy storage technologies will evolve, and green hydrogen will see more extensive application
Sectors like industry and transportation are the main sources of carbon emissions through energy consumption. To reduce emissions, priority should be given to green transformation in traditional industrial sectors by promoting green electricity and electric manufacturing. We should focus on optimizing transportation structures, promoting green mobility, and constructing more renewable energy infrastructure. Additionally, applying technologies such as smart grids, 5G, and AI will help reduce carbon emissions and contribute to the development of green, low-carbon cities.
Besides, energy storage systems (ESSs) can store electric energy during off-peak hours and discharge that energy during peak hours for peak shaving and load balancing, thus improving the operating efficiency and reliability of power grids while cutting power system investment. Various new energy storage technologies, such as compressed-air energy storage, electrochemical energy storage, and thermal (cold) energy storage, will coexist to meet system regulation requirements.
New technologies and business models, such as hydrogen metallurgy, hydrogen production from renewables, ammonia/methanol synthesis by green hydrogen, and hydrogen-based power generation, will all be widely promoted. Electricity will interact with secondary energy sources like hydrogen through electricity-to-hydrogen conversion and electric fuel production, helping build a multi-energy complementary system that interconnects multiple energy sources with electric energy. In fields such as metallurgy, chemical industry, transportation, and power generation, hydrogen, as a reacting substance or raw material, will become essential to clean electricity.
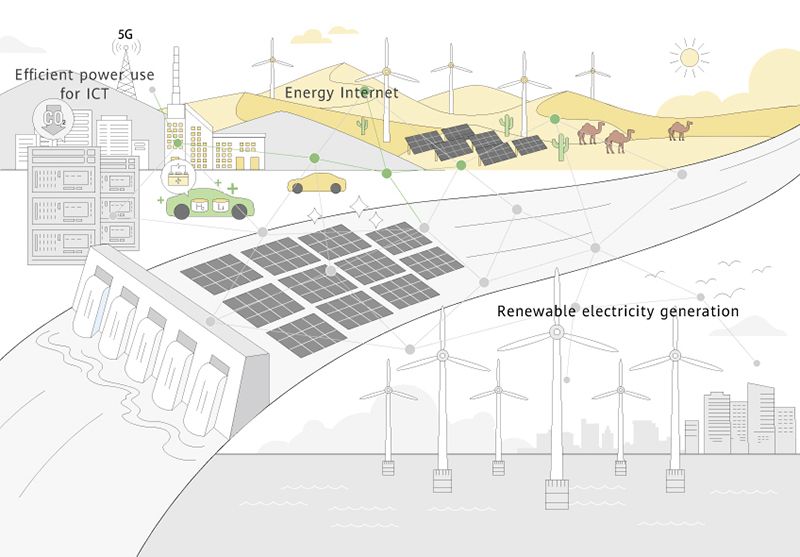
Intelligent generation-grid-load-storage-consumption through the Energy Internet
Snapshot from the future: Virtual power plants, a paradigm shift for the power value chain
The emergence of virtual power plants (VPPs) is redrawing the boundaries between power producers and power consumers. VPPs are set to reshape the power generation value chain.
VPPs will leverage economies of scale to realize the commercial model that distributed energy producers cannot achieve alone. In order to participate in the future energy market and generate profits, distributed energy producers should be able to sense market prices in real time. Distributed new energy devices will need to respond to market changes and power grid fluctuations in real time. This requires ICT infrastructure such as interconnected networks and edge gateways or edge computing. Producers will incur the kinds of transaction costs that come with being part of the market, such as insurance and compliance costs. These additional costs represent a barrier to market entry for distributed energy producers, but by aggregating the large number of distributed energy sources, VPPs reduce costs and generate profits through economies of scale.
Energy cloud can be understood as the operating system of the energy Internet, and is typically characterized by convergence, openness, and intelligence.
Generation, grids, storage, and consumption of power need to be converged in an end-to-end manner. Generators now include a large number of distributed new energy sources, such as solar energy, wind energy, and biomass, as well as fossil fuel sources such as gas.
In addition, the energy cloud needs to interconnect with third-party systems, such as carbon trading systems. Therefore, the energy cloud should be an open ecosystem.
To enable convergence and openness, the energy cloud must be an intelligent platform. With the support of efficient, intelligent technologies such as AI and big data, the energy cloud aims to enable a frictionless flow of energy from producers to consumers as they demand it. Ultimately, it will create a green, low-carbon, safe, stable, and diverse energy system.
As generation-grid-storage-load synergy accelerates and deepens, the boundaries of the traditional value chain will be broken, and power systems will no longer adjust electricity generation simply based on plans and loads. Instead, electricity supply and demand will become more flexible and dynamic. To drive the digital and intelligent transformation of the new power system, next-generation technologies will be vigorously developed and extensively applied in areas such as digital edges, ubiquitous communications networks, compute and storage, and AI algorithms and applications. The physical world and digital space will be fully connected, device information and production processes in power systems will be converted into digital expressions, and digital mirrors of power systems will be built within the virtual space.
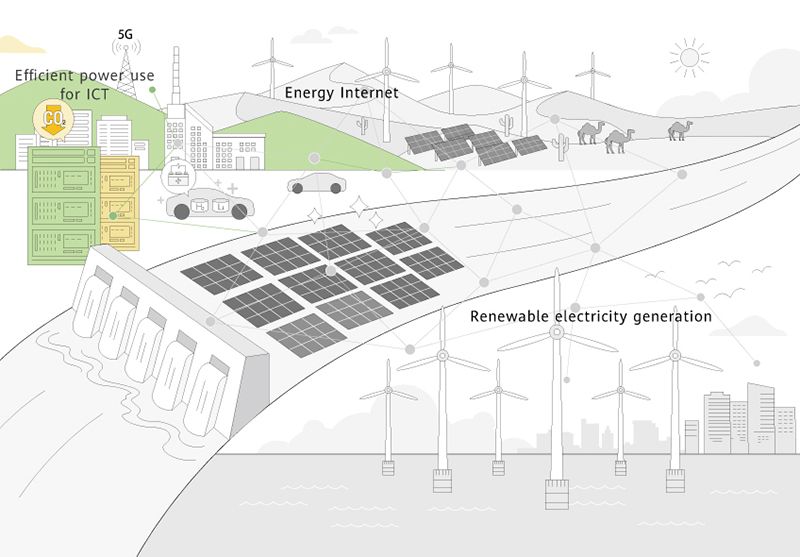
Efficient power use for ICT: Saving energy and cutting more emissions
Snapshot from the future: Low-carbon data centers and sites
The ICT industry needs to cut emissions and save energy while enabling other industries to reduce carbon emissions. Data centers and telecom networks are the primary sources of carbon emissions in the ICT industry.
Data centers reduce carbon emissions by purchasing green power and applying free cooling and AI. Large ICT companies have been the biggest purchasers of green power, as they strive to reduce carbon emissions in data centers and telecoms networks.
As an increasing number of high-temperature-proof servers are put into use, cooling using natural air instead of traditional chillers and air conditioners will become possible. This will reduce the energy consumption of cooling systems, thereby decreasing the PUE.
In addition to applying renewable energy and free cooling, AI is another effective way to make data centers more efficient and save energy. Sensors in data centers collect data such as temperature, power levels, pump speed, power consumption rate, and settings, which are analyzed using AI. Then, the data center operations and control thresholds are adjusted accordingly, reducing costs and increasing efficiency.
The green telecoms networks of the future will be built to support more than 100 times today's capacity, but their total energy consumption will be no higher than that of today's networks. Conventional telecoms networks are defined by their specialist functions, which makes for fragmented operation and maintenance (O&M) and means they cannot keep pace with the latest network automation and intelligence. Networks need to be reconstructed to deliver essential services through a simplified architecture that consists of three layers: basic telecoms network, cloud network, and algorithms. This simplified network architecture will greatly reduce the complexity of the algorithms in autonomous driving networks, reducing the demand for computing, and cut O&M costs, contributing to greener, low-carbon networks.
More Outlooks
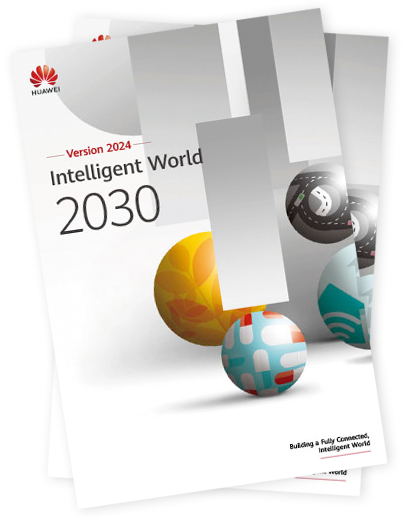